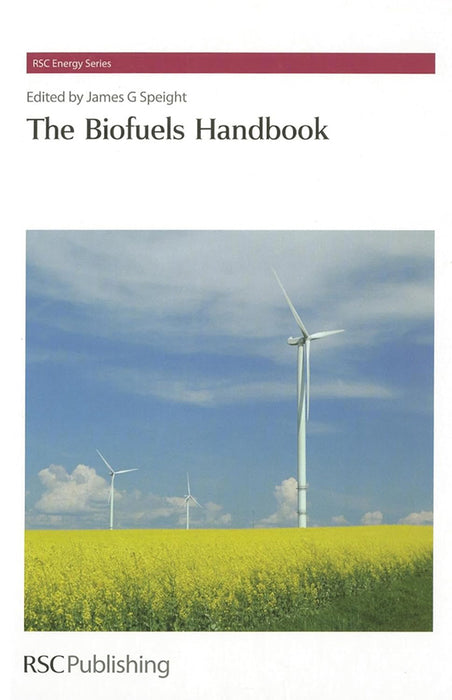
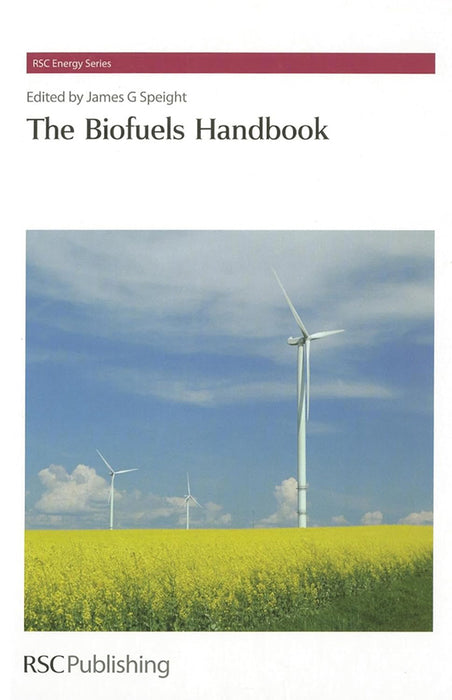
Petroleum-based fuels are well-established products that have served industry and consumers for more than one hundred years. However petroleum, once considered inexhaustible, is now being depleted at a rapid rate. As the amount of available petroleum decreases, the need for alternative technologies to produce liquid fuels that could potentially help prolong the liquid fuels culture and mitigate the forthcoming effects of the shortage of transportation fuels is being sought. The dynamics are now coming into place for the establishment of a synthetic fuels industry; the processes for recovery of raw materials and processing options have to change to increase the efficiency of oil production and it is up to various levels of government not only to promote the establishment of such an industry but to recognise the need for available and variable technology. This timely handbook is written to assist the reader in understanding the options that available for the production of synthetic fuel from biological sources. Each chapter contains tables of the chemical and physical properties of the fuels and fuel sources. It is essential that the properties of such materials be presented in order to assist the researcher to understand the nature of the feedstocks as well as the nature of the products. If a product cannot be employed for its hope-for-use, it is not a desirable product and must be changed accordingly. Such plans can only be made when the properties of the original product are understood. The fuels considered include conventional and unconventional fuel sources; the production and properties of fuels from biomass, crops, wood, domestic and industrial waste and landfill gas.
Speight has more than forty years of experience in areas associated with (1) the properties and recovery of reservoir fluids, including heavy oil, and tar sand bitumen, (2) refining conventional petroleum as well as heavy oil, tar sand bitumen, synthetic fuels, and biofuels, (3) the properties of fuels, synthetic fuels, and biofuels, (4) the properties, behavior, and processing of natural gas, including gas-to-liquids, (5) the properties and behavior of coal, including coal liquids, and (6) the properties and behavior of oil shale, including shale oil. Environmental effects and remediation technologies related to fossil fuel and synthetic fuel processing. He is the author of more than four hundred publications, reports, and presentations and more than thirty books and bibliographies related to fossil fuels, synthetic fuels, biofuels, fuels processing, and environmental issues. Dr Speight is visiting Professor at University of Utah, University of Trinidad and Tobago. Technical University of Denmark (Lyngby, Denmark), University of Petroleum (Beijing, China), University of Regina (Regina, Saskatchewan, Canada), and University of Akron (Akron, Ohio, USA).
Excerpt. © Reprinted by permission. All rights reserved.
The Biofuels Handbook
By James G. Speight
The Royal Society of Chemistry
Copyright © 2011 The Royal Society of Chemistry
All rights reserved.
ISBN: 978-1-84973-026-6
Contents
Part 1 Fuels from Conventional and Unconventional Sources,
Chapter 1 Conventional Fuel Sources James G. Speight, 3,
Chapter 2 Unconventional Fuel Sources James G. Speight, 42,
Chapter 3 Fuels from Biomass – Overview Mustafa Balat, 76,
Chapter 4 A Biorefinery James G. Speight, 118,
Chapter 5 Biofuels Natasha Ramroop Singh, 160,
Part 2 Fuels from Cellulosic and Lignocellulosic Materials,
Chapter 1 Production of Fuels from Crops Ayhan Demirbas, 201,
Chapter 2 Properties of Fuels from Crops Ayhan Demirbas, 228,
Chapter 3 Production of Fuels from Wood Sources Mrinal K. Ghose, 255,
Chapter 4 Properties of Fuels from Wood Sources James G. Speight, 304,
Part 3 Fuels from Waste Materials,
Chapter 1 Production and Properties of Fuels from Domestic and Industrial Waste Ejae John and Kamel Singh, 333,
Chapter 2 Properties of Fuels from Domestic and Industrial Waste Ejae John and Kamel Singh, 377,
Chapter 3 Production of Fuels from Landfills Kamel Singh and Musti K.S. Sastry, 408,
Chapter 4 Uses of Landfill Gas Solange Kelly, 454,
Chapter 5 The Fischer–Tropsch Process Ralph Chadeesingh, 476,
Glossary, 518,
Subject Index, 542,
CHAPTER 1
Part 1
Fuels from Conventional and Unconventional Sources
CHAPTER 1
Conventional Fuel Sources
JAMES G. SPEIGHT
CD&W Inc., PO Box 1722, Laramie, WY 82070-4808, USA
1.1 Introduction
Conventional fuel sources are the two major hydrocarbon natural products – petroleum and natural gas. Both petroleum and natural gas are fossil fuels and are not replenished rapidly.
Nonconventional fuels (alternative fuels) are any materials or substances that can be used as fuels, other than conventional fuels. Examples of nonconventional fuels include biodiesel, bioalcohol (methanol, ethanol, butanol), hydrogen, and fuels from biomass sources. In fact, a biofuel is any as solid, liquid, or gaseous fuel consisting of, or derived from biomass. Biomass can also be used directly for heating or power — known as biomass fuel. Biofuel can be produced from any carbon source that can be replenished rapidly, e.g. plants. Many different plants and plant-derived materials are used for biofuel manufacture.
Petroleum (also called crude oil) also includes crude oil, natural gas, and heavy oil (a type of petroleum). Tar sand bitumen is not included because it is not a type of petroleum (Speight, 2007). Both crude oil and natural gas are predominantly a mixture of hydrocarbons. Under conditions of standard temperature and pressure at the surface, the lower molecular weight hydrocarbons methane, ethane, propane, and butane occur as gases, while the higher molecular weight hydrocarbons are in the form of liquids and/or solids.
An oil well produces predominantly petroleum and natural gas and, because the pressure is lower at the surface than it is in the underground formation (reservoir), some of the gas will come out of solution and be recovered (associated gas, solution gas).
On the other hand, a gas well produces predominately natural gas, which is a gaseous fossil fuel consisting primarily of methane but including significant quantities of ethane, butane, propane, carbon dioxide, nitrogen, helium, and hydrogen sulfide. It is found in natural gas fields (unassociated natural gas, nonassociated natural gas), oil fields (associated natural gas) and in coal seams or coal beds (coalbed methane).
However, because the underground temperature and pressure are higher than at the surface, the gas may contain heavier hydrocarbons such as pentane, hexane, heptane, and octane in the gaseous state. Under surface conditions these will condense out of the gas (natural gas condensate,condensate) and the condensed liquid resembles gasoline in appearance and is similar in composition to light crude oil.
Unprocessed petroleum and natural gas are not generally useful and are sent (by pipeline and/or by ocean tanker) to a refinery where the different hydrocarbon molecules are separated into the various components that can be used as fuels, lubricants, road asphalt, and as feedstock for petrochemical processes that manufacture such products as plastics, detergents, solvents, elastomers, and fibers such as nylon and polyesters. Petroleum refining is the means by which crude petroleum is converted to a series of saleable products.
A petroleum refinery is a group of manufacturing plants (Figure 1.1) which are used to separate petroleum into fractions and the subsequent treating of these fractions to yield marketable products, particularly fuels (Kobe and McKetta, 1958; Nelson, 1958; Gruse and Stevens, 1960; Bland and Davidson, 1967; Hobson and Pohl, 1973; Speight, 2007). The configuration of refineries may vary from refinery to refinery. Some refineries may be more oriented toward the production of gasoline (reforming and/or catalytic cracking) whereas the configuration of other refineries may be more oriented towards the production of middle distillates such as jet fuel, and gas oil.
In general, crude oil, once refined, yields three basic groupings of products that are produced when it is broken down into cuts or fractions (Table 1.1). The gas and gasoline cuts form the lower boiling products and are usually more valuable than the higher-boiling fractions and provide gas (liquefied petroleum gas), naphtha, aviation fuel, motor fuel and feedstocks for the petrochemical industry. Naphtha, a precursor to gasoline and solvents, is extracted from both the light and middle range of distillate cuts and is also used as a feedstock for the petrochemical industry. The middle distillates refer to products from the middle boiling range of petroleum and include kerosene, diesel fuel, distillate fuel oil, and light gas oil. Waxy distillate and lower boiling lubricating oils are sometimes included in the middle distillates. The remainder of the crude oil includes the higher-boiling lubricating oils, gas oil, and residuum (the nonvolatile fraction of the crude oil). The residuum can also produce heavy lubricating oils and waxes but is more often used for asphalt production. The complexity of petroleum is emphasized insofar as the actual proportions of light, medium and heavy fractions vary significantly from one crude oil to another.
The yields and quality of refined petroleum products produced by any given oil refinery depend on the mixture of crude oil used as feedstock and the configuration of the refinery facilities. Light/sweet crude oil is generally more expensive and has inherent high yields of higher-value low-boiling products such as naphtha, gasoline, jet fuel, kerosene, and diesel fuel. Heavy sour crude oil is generally less expensive and produces greater yields of lower-value higher-boiling products that must be converted into lower boiling products.
This chapter presents an overview of petroleum refining in order for the reader to place each process in the correct context of the production of conventional fuels.
1.2 Petroleum Refining
1.2.1 Dewatering and Desalting
Petroleum is recovered from the reservoir mixed with a variety of substances: gases, water, and dirt (minerals). Thus, refining actually commences with the production of fluids from the well or reservoir and is followed by pretreatment operations that are applied to the crude oil either at the refinery or prior to transportation. Pipeline operators, for instance, are insistent upon the quality of the fluids put into the pipelines; therefore, any crude oil to be shipped by pipeline or, for that matter, by any other form of transportation must meet rigid specifications in regard to water and salt content. In some instances, sulfur content, nitrogen content, and viscosity may also be specified.
Field separation, which occurs at a field site near the recovery operation, is the first attempt to remove the gases, water, and dirt that accompany crude oil coming from the ground. The separator may be no more than a large vessel that gives a quieting zone for gravity separation into three phases: gases, crude oil, and water containing entrained dirt.
Desalting is a water-washing operation performed at the production field and at the refinery site for additional crude oil cleanup (Figure 1.2). If the petroleum from the separators contains water and dirt, water washing can remove much of the water-soluble minerals and entrained solids. If these crude-oil contaminants are not removed, they can cause operating problems during refinery processing, such as equipment plugging and corrosion as well as catalyst deactivation.
1.2.2 Distillation
Distillation was the first method by which petroleum was refined. In the early stages of refinery development, when illuminating and lubricating oils were the main products, distillation was the major and often only refinery process. At that time gasoline was a minor, but more often unwanted, product. As the demand for gasoline increased, conversion processes were developed because distillation could no longer supply the necessary quantities of this volatile product.
It is possible to obtain fuels ranging from gaseous materials taken off at the top of the distillation column to a nonvolatile residue or reduced crude (bottoms), with correspondingly lighter materials at intermediate points. The reduced crude may then be processed by vacuum, or steam, distillation in order to separate the high-boiling lubricating oil fractions without the danger of decomposition, which occurs at high (>350 °C, >660 °F) temperatures. Atmospheric distillation may be terminated with a lower-boiling fraction (cut) if it is felt that vacuum or steam distillation will yield a better-quality product, or if the process appears to be economically more favorable. Not all crude oils yield the same distillation products and the nature of the crude oil dictates the processes that may be required for refining.
1.2.2.1 Atmospheric Distillation
The distillation unit is a collection of distillation units but, in contrast to the early battery units, a tower is used in the modern-day refinery (Figure 1.3) and brings about an efficient degree of fractionation (separation).
The feed to a distillation tower is heated by flow through pipes arranged within a large furnace. The heating unit is known as a pipe still heater or pipe still furnace, and the heating unit and the fractional distillation tower make up the essential parts of a distillation unit or pipe still. The pipe still furnace heats the feed to a predetermined temperature – usually a temperature at which a predetermined portion of the feed will change into vapor. The vapor is held under pressure in the pipe in the furnace until it discharges as a foaming stream into the fractional distillation tower. Here, the unvaporized or liquid portion of the feed descends to the bottom of the tower to be pumped away as a bottom nonvolatile product, while the vapors pass up the tower to be fractionated into gas oils, kerosene, and naphtha.
Pipe still furnaces vary greatly and, in contrast to the early units where capacity was usually 200 to 500 bbl per day, can accommodate 25 000 bbl, or more of crude petroleum per day. The walls and ceiling are insulated with firebrick and the interior of the furnace is partially divided into two sections: a smaller convection section where the oil first enters the furnace and a larger section (fitted with heaters) where the oil reaches its highest temperature.
All of the primary fractions from a distillation unit are equilibrium mixtures and contain some proportion of the lighter constituents characteristic of a lower-boiling fraction. The primary fractions are stripped of these constituents (stabilized) before storage or further processing.
1.2.2.2 Vacuum Distillation
Vacuum distillation as applied to the petroleum refining industry evolved because of the need to separate the less-volatile products, such as lubricating oils, from the petroleum without subjecting these high-boiling products to cracking conditions. The boiling point of the heaviest cut obtainable at atmospheric pressure is limited by the temperature (ca. 350 °C; ca. 660 °F) at which the residue starts to decompose (crack). When the feedstock is required for the manufacture of lubricating oils, further fractionation without cracking is desirable and this can be achieved by distillation under vacuum conditions.
Operating conditions for vacuum distillation (Figure 1.4) are usually 50 to 100 mm of mercury (atmospheric pressure = 760 mm of mercury). In order to minimize large fluctuations in pressure in the vacuum tower, the units are necessarily of a larger diameter than the atmospheric units. Some vacuum distillation units have diameters on the order of 45 ft (14 m). By this means, a heavy gas oil may be obtained as an overhead product at temperatures of about 150 °C (300 °F), and lubricating oil cuts may be obtained at temperatures of 250 to 350 °C (480 to 660 °F), feed and residue temperatures being kept below the temperature of 350 °C (660 °F), above which cracking will occur. The partial pressure of the hydrocarbons is effectively reduced still further by the injection of steam. The steam added to the column, principally for the stripping of asphalt in the base of the column, is superheated in the convection section of the heater.
The fractions obtained by vacuum distillation of the reduced crude (atmospheric residuum) from an atmospheric distillation unit depend on whether or not the unit is designed to produce lubricating or vacuum gas oils. In the former case, the fractions include (1) heavy gas oil, which is an overhead product and is used as catalytic cracking stock or, after suitable treatment, a light lubricating oil, (2) lubricating oil (usually three fractions – light, intermediate, and heavy), which is obtained as a side-stream product, and (3) asphalt (or residuum), which is the bottom product and may be used directly as, or to produce, asphalt and that may also be blended with gas oils to produce a heavy fuel oil.
1.2.3 Thermal Processes
Cracking distillation (thermal decomposition with simultaneous removal of distillate) was recognized as a means of producing the valuable lighter product (kerosene) from heavier nonvolatile materials. In the early days of the process (1870 to 1900) the technique was very simple – a batch of crude oil was heated until most of the kerosene had been distilled from it and the overhead material had become dark in color. At this point distillation was discontinued and the heavy oils were held in the hot zone, during which time some of the high molecular weight components were decomposed to produce lower molecular weight products. After a suitable time, distillation was continued to yield light oil (kerosene) instead of the heavy oil that would otherwise have been produced.
1.2.3.1 Thermal Cracking
One of the earliest conversion processes used in the petroleum industry is the thermal decomposition of higher-boiling materials into lower-boiling products. The heavier oils produced by cracking are light and heavy gas oils as well as a residual oil that could also be used as heavy fuel oil. Gas oils from catalytic cracking were suitable for domestic and industrial fuel oils or as diesel fuels when blended with straight-run gas oils. The gas oils produced by cracking were also a further important source of gasoline. In a once-through cracking operation all of the cracked material is separated into products and may be used as such. However, the gas oils produced by cracking (cracked gas oils) are more resistant to cracking (more refractory) than gas oils produced by distillation (straight-run gas oils) but could still be cracked to produce more gasoline. This was achieved using a later innovation (post-1940) involving a recycle operation in which the cracked gas oil was combined with fresh feed for another trip through the cracking unit. The extent to which recycling was carried out affected the yield of gasoline from the process.
The majority of the thermal cracking processes use temperatures of 455 to 540 °C (850 to 1005 °F) and pressures of 100 to 1000 psi; the Dubbs process may be taken as a typical application of an early thermal cracking operation. The feedstock (reduced crude) is preheated by direct exchange with the cracking products in the fractionating columns. Cracked gasoline and heating oil are removed from the upper section of the column. Light and heavy distillate fractions are removed from the lower section and are pumped to separate heaters. Higher temperatures are used to crack the more refractory light distillate fraction. The streams from the heaters are combined and sent to a soaking chamber where additional time is provided to complete the cracking reactions. The cracked products are then separated in a low-pressure flash chamber where a heavy fuel oil is removed as bottoms. The remaining cracked products are sent to the fractionating columns.
(Continues...)
Excerpted from
The Biofuels Handbook
by
James G. Speight
. Copyright © 2011 The Royal Society of Chemistry. Excerpted by permission of The Royal Society of Chemistry.
All rights reserved. No part of this excerpt may be reproduced or reprinted without permission in writing from the publisher.
Excerpts are provided by Dial-A-Book Inc. solely for the personal use of visitors to this web site.
Read less
- Publisher: Royal Society of Chemistry
- Publisher Imprint:
- Publication Date:
- Pages: 574
- ISBN13: 9781849730266
- Item Weight: 1050 grams
- Original Price: 280.0 GBP
- Edition: N/A
- Binding: Hardcover