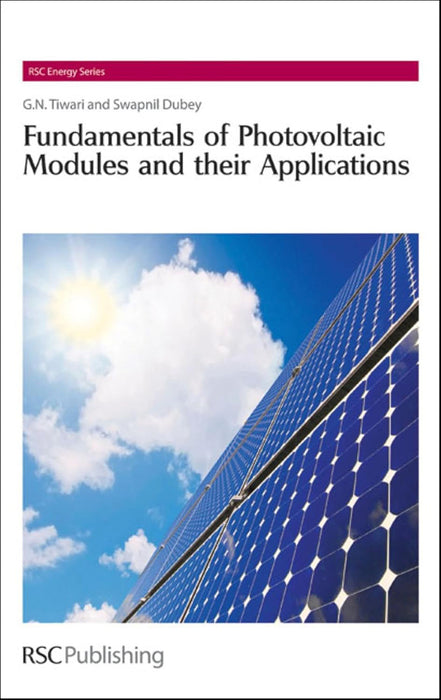
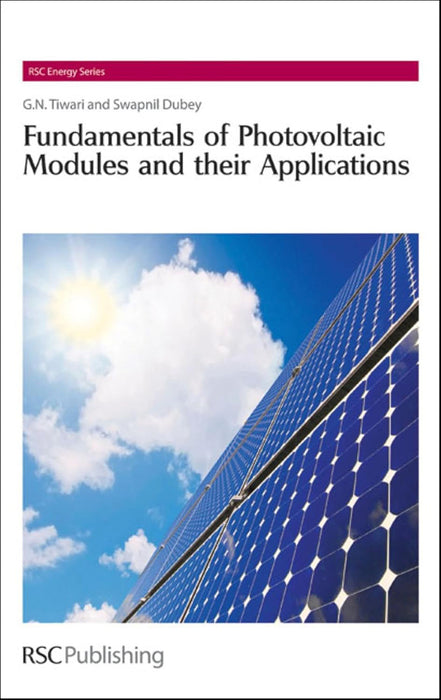
The first book to cover the fundamentals of solar energy, photovoltaic or photovoltaic thermal technologies, energy security and climate change.
ofessor Gopal Nath Tiwari, born on July 01, 1951 at Adarsh Nagar, Sagerpali, Ballia (UP), India. He had received postgraduate and doctoral degrees in 1972 and 1976, respectively, from Banaras Hindu University (B.H.U.). Over several years since 1977, He has been actively involved in the teaching programme at Centre for Energy Studies, IIT Delhi. His research interest in the field of Solar Thermal Applications are solar distillation, water/air heating system, greenhouse technology for agriculture as well as for aquaculture, Earth to air heat exchanger, passive building design and hybrid photovoltaic thermal (HPVT) systems. He has guided about 50 Ph.D. students and published over 400 research papers in journals of repute. He has authored eleven books associated with reputed publishers namely Pergaman Press UK, CRC Press USA, Narosa Publishing House etc. He is a co-recipient of 'Hariom Ashram Prerit S.S. Bhatnagar' Award in 1982. Professor Tiwari has been recognized both at national and international levels. His contribution for successful implementation of hot water system in the IIT campus has been highly appreciated. He had been to the University of Papua, New Guinea in 1987-1989 as Energy and Environment Expert. He was also a recipient of European Fellow in 1997 and been to the University of Ulster (U.K.) in 1993. Besides, he had been nominated for IDEA award in the past. He is responsible for development of Solar Energy Park at IIT Delhi and Energy Laboratory at University of Papua, New Guinea, Port Moresby. Dr. Tiwari had visited many countries namely Italy, Canada, USA, UK, Australia, Greece, Thailand, Singapore , PNG and Taiwan etc. for invited talks, chairing international conferences, expert in renewable energy, presenting research papers etc. He has successfully co-coordinated various research projects on Solar distillation, water heating system, Greenhouse technology, hybrid photovoltaic thermal (HPVT) etc. funded by Govt. of India in recent past. Recentl
Mr. Swapnil Dubey was born on July 20, 1981 at Indore (M.P.). He had received Bachelor of Engineering degree in Mechanical Engg. from Institute of Engineering and Technology, Devi Ahilya Vishwavidyalaya, Indore in 2004. He received post graduation degree (M. Tech.) in Energy Studies from Centre for Energy Studies, Indian Institute of Technology (IIT) Delhi in 2006. His M. Tech. project was on 'Performance Evaluation of a Symmetrical Double Slope Passive Solar Distillation Unit' under Prof. G. N. Tiwari. Based on M. Tech. project he had presented two papers in international conferences, viz, International Symposium on 'Desalination and Water purification: Water Resources and their Management' held at MNIT Jaipur during March 20-21, 2006 and 3rd International Conference on 'Solar Radiation and Day Lighting' SOLARIS 2007 held at IIT Delhi during February 7-9, 2007. Presently, he is pursuing Ph.D. under the supervision of Prof. G. N. Tiwari from Centre for Energy Studies, IIT Delhi. The topic of his research work is 'Performance Evaluation of Hybrid Photovoltaic thermal (PV/T) solar water heater'. Based on his research work he has communicated four papers in international journals, viz, Solar Energy, Solar Energy Materials and Solar Cells, Energy Research and Renewable Energy. During Ph.D., he has also worked as an organizing member in 3rd International Conference on 'Solar Radiation and Day Lighting' SOLARIS 2007 held at IIT Delhi during February 7-9, 2007 He is expertise in the field of solar distillation, solar thermal, photovoltaic, exergy analysis and carbon trading.
Excerpt. © Reprinted by permission. All rights reserved.
Fundamentals of Photovoltaic Modules and Their Applications
By G. N. Tiwari, Swapnil Dubey
The Royal Society of Chemistry
Copyright © 2010 G. N. Tiwari and Swapnil Dubey
All rights reserved.
ISBN: 978-1-84973-020-4
Contents
Chapter 1 Solar Radiation, 1,
Chapter 2 History of PV-integrated Systems, 29,
Chapter 3 Solar Cell Materials and Their Characteristics, 81,
Chapter 4 PV Array Analysis, 110,
Chapter 5 Role of Batteries and Their Uses, 130,
Chapter 6 Case Studies of PV/T Systems, 157,
Chapter 7 Thermal Modelling of Hybrid Photovoltaic/Thermal (PV/T) Systems, 174,
Chapter 8 Energy and Exergy Analysis, 257,
Chapter 9 CO2 Mitigation and Carbon Trading, 302,
Chapter 10 Economic Analysis, 327,
Appendix I, 369,
Appendix II, 373,
Appendix III, 379,
Appendix IV, 381,
Appendix V, 385,
Appendix VI, 387,
Glossary, 388,
Subject Index, 398,
CHAPTER 1
Solar Radiation
1.1 Introduction
Sunlight, in the broad sense, is the total spectrum of the electromagnetic radiation given off by the Sun. On Earth, sunlight is filtered through the atmosphere, and the solar radiation is obvious as daylight when the Sun is above the horizon. This is usually during the day hours. Near the poles in summer, sunlight also occurs during the night hours and in the winter at the poles sunlight may not occur at any time. When the direct radiation is not blocked by clouds, it is experienced as sunshine, a combination of bright light and heat. Radiant heat directly produced by the radiation of the Sun is different from the increase in atmospheric temperature due to the radiative heating of the atmosphere by the Sun's radiation. Sunlight may be recorded using a sunshine recorder, pyranometer or pyrheliometer. The World Meteorological Organization (WMO) defines sunshine as direct irradiance from the Sun measured on the ground of at least 120 Wm-2. Direct sunlight gives about 93 lux of illumination per watt of electromagnetic power, including infrared, visible and ultraviolet. Bright sunlight provides illumination of approximately 100 000 lux per square metre at the Earth's surface. Sunlight is a key factor in the process of photosynthesis.
1.1.1 The Sun
The Sun is the star at the centre of the solar system. The Earth and other matter (including other planets, asteroids, meteoroids, comets and dust) orbit the Sun, which by itself accounts for about 99.8% of the solar system's mass. Energy from the Sun, in the form of sunlight, supports almost all life on Earth via photosynthesis, and drives the Earth's climate and weather.
The Sun has an effective black-body temperature TS of 5777 K and it is the largest member of the solar system. The Sun is a sphere of intensely hot, gaseous matter with a diameter of 1.39 x 109 m and is, on average, 1.5 x 1011 m away fromthe Earth. The Sun is, effectively, a continuous fusion reactor. It is estimated that 90% of the Sun's energy is generated in the region 0 to 0.23 R (R being the radius of the Sun = 6.95x 108 m); the average density (ρ) and the temperature (T) in this region are 105 kgm-3 and about 8-40 x 106 K respectively. At a distance of about 0.7 R from the centre, the temperature drops to about 1.3 x 105 K and the density to 70 kg m-3. Hence for r > 0.7R convection begins to be important and the region 0.7RR is known as the convective zone. The outer layer of this zone is called the photosphere. The maximum spectral intensity occurs at about 0.48 mm wavelength λ in the green portion of the visible spectrum. About 8.73% of the total energy is contained in the ultraviolet region (λ<0.40 µm); another 38.15% in the visible region (0.40 µm0.70 µm).
1.1.2 The Earth
Earth is the third planet from the Sun. Earth is the largest of the terrestrial planets in the solar system in diameter, mass and density. The Earth, almost round in shape with a diameter of about 13 000 km, came into existence some 4.6 x 109 years ago. The Earth's inner core is a solid made of iron and nickel. The eruption of volcanoes generally occurs at the plate boundary of the Earth. During eruption of volcanoes, various greenhouse gases, namely carbon dioxide (CO2), methane (CH4), nitrous oxide (NOx), ozone (O3) and water vapour (H2O) etc., existing inside the ground, are also discharged through the plate boundary. These discharged gases, at the boundary of the plate, move upwards towards the Sun due to its low density. These gases form a layer between the Sun and Earth (Figure 1.1). This layer is generally referred to as the Earth's atmosphere. The Earth revolves around the Sun once in about a year. Nearly two-thirds of the Earth is covered by water and the remaining one-third is land. Half of the Earth is lit by sunlight at a time. It reflects one-third of the sunlight that falls on it. This is known as Earth's albedo. The Earth is spinning at a constant rate about its axis, inclined at an angle of 23.5°. As a result, the lengths of days and nights are constantly changing. The heat flux at Earth's surface due to heat conduction from the centre is 0.04–0.06 W m-2 with a temperature gradient of 30–40°C km-1.
1.1.3 Earth's Atmosphere
The temperature of the Earth's atmosphere varies with altitude among five different atmospheric layers:
Exosphere: from 500–1000 km up to 10 000 km, free-moving particles that may migrate into and out of the magnetosphere or the solar wind.
Ionosphere: the part of the atmosphere that is ionized by solar radiation. It plays an important part in atmospheric electricity and forms the inner edge of the magnetosphere. It has practical importance because, among other functions, it influences radio propagation to distant places on the Earth. It is located in the thermosphere and is responsible for auroras.
Thermosphere: from 80–85 km to 640 + km, the temperature increasing with height.
Mesosphere: extends from about 50 km to the range of 80–85 km, the temperature decreasing with height. This is also where most meteors burn up when entering the atmosphere.
Stratosphere: extends from the troposphere's 7- to 17-km range to about 50 km. Temperature increases with height. The stratosphere contains the ozone layer, the part of the Earth's atmosphere which contains relatively high concentrations of ozone. 'Relatively high' means a few parts per million (ppm) – much higher than the concentrations in the lower atmosphere but still small compared to the main components of the atmosphere. It is mainly located in the lower portion of the stratosphere from approximately 15 to 35 km above Earth's surface, though the thickness varies seasonally and geographically.
Troposphere: the lowest layer of the atmosphere; it begins at the surface and extends to between 7 km at the poles and 17 km at the equator, with some variation due to weather factors. The troposphere has a great deal of vertical mixing because of solar heating at the surface. This heating warms air masses, which makes them less dense so they rise. When an air mass rises, the pressure upon it decreases so it expands, doing work against the opposing pressure of the surrounding air. To do work is to expend energy, so the temperature of the air mass decreases. As the temperature decreases, water vapour in the air mass may condense or solidify, releasing latent heat that further uplifts the air mass. This process determines the maximum rate of decline of temperature with height, called the adiabatic lapse rate. The troposphere contains roughly 80% of the total mass of the atmosphere. Fifty percent of the total mass of the atmosphere is located in the lower 5.6 km of the troposphere.
The average temperature of the atmosphere at the surface of Earth is 15 °C. The average atmospheric pressure, at sea level, is about 101.3 kilopascals with a scale height of about 8.5km; total atmospheric mass is 5.1480xl018 kg. Atmospheric pressure is a direct result of the total weight of the air above the point at which the pressure is measured. This means that air pressure varies with location and time, because the amount (and weight) of air above the Earth varies with location and time.
The density of air at sea level is about 1.2kgm-3. Natural variations of the barometric pressure occur at any one altitude as a consequence of weather. The atmospheric density decreases as the altitude increases. This variation can be approximately modelled using the barometric formula. More sophisticated models are used by meteorologists and space agencies to predict weather and orbital decay of satellites.
Solar radiations while passing through the Earth's atmosphere are subjected to the mechanisms of atmospheric absorption and scattering. The X-rays and extreme ultraviolet radiations of the Sun are highly absorbed in the ionosphere by nitrogen, oxygen and other atmospheric gases. The ozone and water vapours largely absorb ultraviolet (λ<0.40 µm) and infrared radiations (λ>2.3 µm). There is almost complete absorption of short wave radiations (λ<0.29 µm) in the atmosphere. Hence, the energy incident on the Earth's surface in wavelength radiation below 0.29 µm and above 2.3 µm of the spectra of the solar radiation is negligible.
The Earth's atmosphere has the following unique properties:
(a) It absorbs the ultraviolet (UV) and far infrared radiation and allows only radiation having wavelength ranging between 0.29 µm and 2.3 µm, known as short wavelength radiation.
(b) It also does not allow radiation having wavelength λ>2.3 µm, known as long wavelength radiation.
The phenomenon of blocking of UV radiation, referred to as the global greenhouse effect, occurred some 420 million years ago and this allowed plants to grow on the Earth. Fossils (remains of blue-green algae and bacteria) from at least 3xlO years ago have been found in rocks and water. Without the greenhouse effect, the Earth would be a frozen planet with an average temperature of about -18 °C (about 0 °F). For survival of living plants on the Earth, there should be a favourable environment (global environment) in the terrestrial region controlled by short wavelength radiation transmitted by the atmosphere.
However, a similar effect is observed by having transparent material over any surface because the transparent material also behaves as the atmosphere with respect to short wavelength radiation. The concept of trapping short wavelength radiation (thermal energy) in an enclosure has many applications, e.g.:
(a) Flat-plate air collector: A device having an insulated blackened flat surface with a transparent glass window above it that works with the micro greenhouse effect.
(b) Solar dryer: A device that uses solar energy for drying applications.
(c) Greenhouse: A microclimate, which can be created by using the transparent glass/plastic house similar to the global greenhouse concept. It can be used for optimum growth of living plants (e.g. flowers, vegetables, etc.) for maximum crop production during season as well as off-season (post-harvest and pre-harvest period) and is generally known as greenhouse technology. The greenhouse can also be used for crop drying for storage purposes.
(d) Photovoltaic (PV device): A device used to convert short wavelength radiation into direct current (dc) electricity etc.
(e) Solar still: Used for desalination of saline water.
Thus, for optimum design of the above systems, elementary knowledge of solar radiation becomes necessary, which is briefly described as follows.
1.2 Measurement of Solar Radiation on Earth's Surface
The solar radiation reaching the Earth's surface through the atmosphere can be classified into two components: beam and diffuse radiation.
Beam radiation (Ib): The solar radiation propagating along the line joining the receiving surface and the Sun. It is also referred to as direct radiation.
Diffuse radiation (Id): The solar radiation scattered by aerosols, dust and molecules. It does not have any unique direction.
Total radiation (It): The sum of the beam and diffuse radiation, sometimes known as global radiation.
The following instruments are commonly used for measurement of solar radiation on Earth's surface.
1.2.1 Pyrheliometer
The pyrheliometer is a broadband instrument that measures the direct (or beam) component of solar radiation at normal incidence. This means the instrument is always aimed directly at the Sun, via a tracking mechanism that continuously follows the Sun. It is sensitive to wavelengths in the band from 280 to 3000 nm (0.284 µm to 0.3 µm). Solar irradiance enters the instrument through a sealed crystal-quartz window and the sunlight is directed onto a thermopile which converts heat to an electrical signal that can be recorded. A calibration factor is applied when converting the mV signal to an equivalent radiant energy flux, measured in watts per square metre.
In this instrument, two identical blackened manganin strips are arranged so that either one can be exposed to radiation at the base of collimating tubes by moving a reversible shutter. Each strip can be electrically heated and each is fitted with a thermocouple. With one strip shaded and one strip exposed to radiation, a current is passed through the shaded strip to heat it to the same temperature as the exposed strip. When there is no difference in temperature, the electrical energy to the shaded strip must equal the solar radiation absorbed by the exposed strip. Solar radiation is then determined by equating the electrical energy to the product of incident solar radiation, strip area and absorptance. Then the position of the shutter is reversed, interchanging the electrical and radiation heating, and the second value is determined. Alternating the shade and the functions of the two strips compensates for minor differences in the strips, such as edge effects and lack of uniformity of electrical heating.
1.2.2 Pyranometer
A pyranometer is a type of actinometer used to measure broadband solar irradiance on a planar surface and is a sensor that is designed to measure the solar radiation flux density (in watts per metre square) from a field of view of 180°.
The working principle of a pyranometer is the same as a pyrheliometer except for the fact that a sensitive surface is exposed to the total beam, diffused and reflected from Earth and surrounding radiation. The sensitive surface consists of a circular, blackened (hot junction) multijunction thermopile whose cold junctions are electrically insulated from the basement. The temperature difference between the hot and cold junctions is a function of the radiation falling on the surface. The sensitive surface is covered by two concentric hemispherical glass domes to shield it from wind and rain. This also reduces the convection currents. A pyranometer, when provided with an occulting disc, measures the diffuse radiation. This disc, or band, blocks the beam radiation from the surface. The standard distance between the glass dome and the shading ring is 0.3 m. It may be noted that pyranometers are calibrated so as to measure the solar radiation on a horizontal surface. Therefore, when tilted, the change in free convection regime within the glass dome may introduce an error in measurement. A photograph of a typical pyranometer is shown in Figure 1.2.
A pyranometer produces voltage, as a function of the incident solar radiation, from the thermopile detectors. A potentiometer is required to detect and record this output. Radiation data usually must be integrated over some period of time, such as an hour or a day. Integration can be done by means of planimetry or an electronic integrator. Pyranometers have also been based on photovoltaic (solar cell) detectors. Silicon cells are the most common for solar energy measurement, although cadmium sulfide and selenium cells have also been used. Silicon solar cells have the property that their light current (approximately equal to the short-circuit current at normal radiation levels) is a linear function of the incident solar radiation. They have the disadvantage that their spectral response is not linear, so instrument calibration is a function of the spectral distribution of the incident radiation.
A typical pyranometer does not require any power to operate and they are frequently used in meteorology, climatology, solar energy studies and building physics. They can be seen in many meteorological stations, often installed horizontally and next to solar panels, and the sensor is mounted in the surface plane of the panel. Pyranometers are standardized according to the ISO 9060 standard, which is also adopted by the World Meteorological Organization (WMO). Calibration is typically done relative to the World Radiometric Reference (WRR). This reference is maintained by World Radiation Centre (WRC) in Davos, Switzerland.
1.2.3 Sunshine Recorder
Sunshine recorders are used to indicate the amount of sunshine at a given location. The results are used to provide information on the climate of an area and some of the fields it is of importance to are science, agriculture and tourism. Traditionally, sunshine recorders are divided into two groups. In the first group the time of the occurrence of the event is provided by the Sun itself and in the second a clock-type device is used to provide the time scale. The older type of recorder required the interpretation of the results by an observer and these may have differed from one person to another. Today, with the use of electronics and computers, it is possible to record the sunshine duration that does not rely on an observer's interpretation. At the same time the newer recorders can also measure the global and diffuse radiation.
(Continues...)
Excerpted from
Fundamentals of Photovoltaic Modules and Their Applications
by
G. N. Tiwari, Swapnil Dubey
. Copyright © 2010 G. N. Tiwari and Swapnil Dubey. Excerpted by permission of The Royal Society of Chemistry.
All rights reserved. No part of this excerpt may be reproduced or reprinted without permission in writing from the publisher.
Excerpts are provided by Dial-A-Book Inc. solely for the personal use of visitors to this web site.
Read less
- Publisher: Royal Society of Chemistry
- Publisher Imprint:
- Publication Date:
- Pages: 424
- ISBN13: 9781849730204
- Item Weight: 780 grams
- Original Price: 145.0 GBP
- Edition: N/A
- Binding: Hardcover